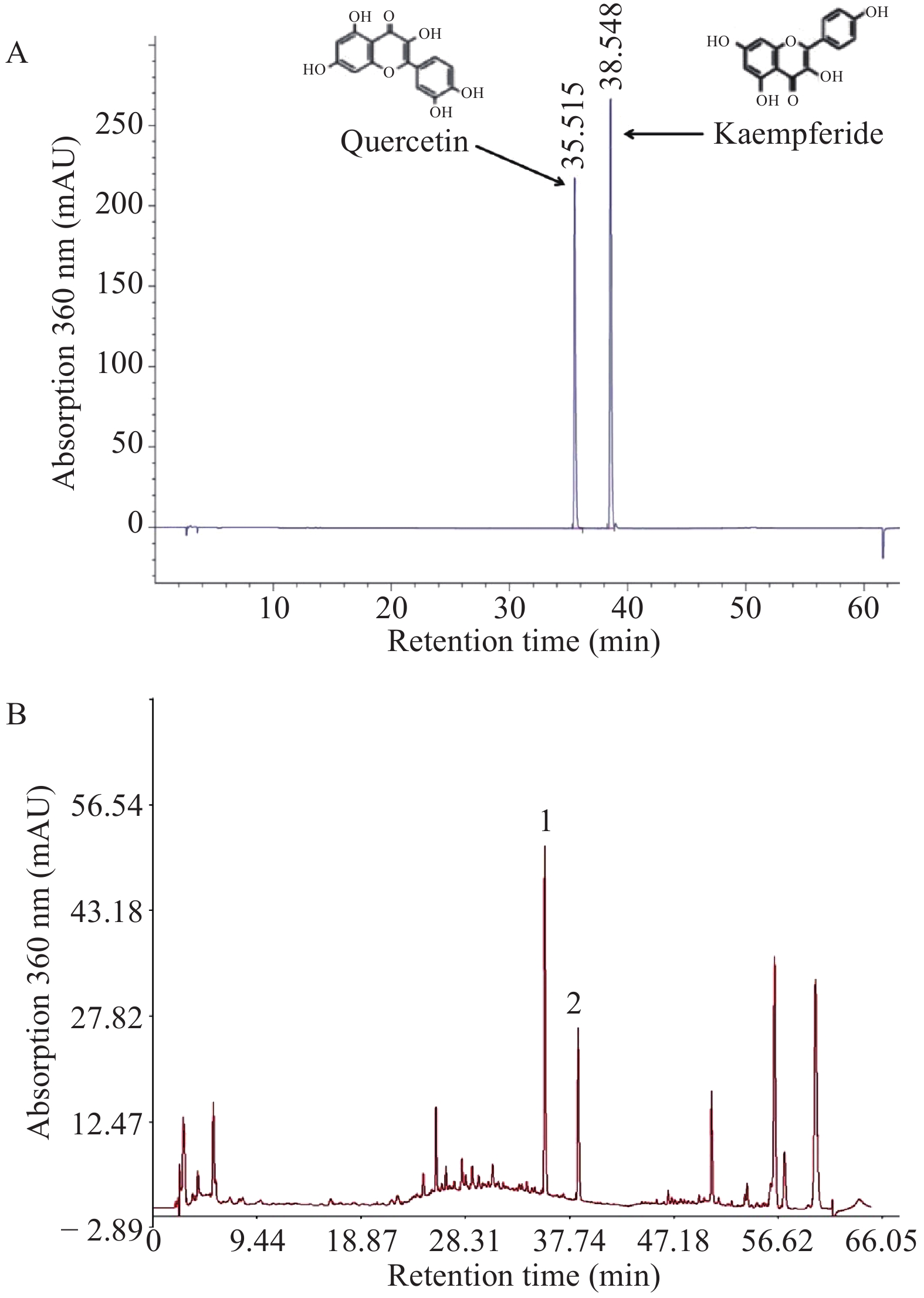
Citation: | WAN Hui-Qi, QI Huan-Yang, XU Guang-Hui, HUANG Yi-Qi. Silkworm Extract Ameliorates Type 2 Diabetes Mellitus and Protects Pancreatic β-cell Functions in Rats[J]. Digital Chinese Medicine, 2020, 3(4): 275-282. DOI: 10.1016/j.dcmed.2020.12.006 |
Silkworm, also known as Bombyx mori, is an economically important insect that feeds on mulberry leaves. Silkworm belongs to the order lepidopteran and the silk moth families, domesticated from the primitive silkworm in ancient China. It is homologous to the wild silkworm, with 28 pairs of chromosomes, found in China today [1]. The silkworm has long been used to treat diabetes in China and Korea; according to the Compendium of Materia Medica (Ben Cao Gang Mu, 《本草纲目》), LI Shi-Zhen, a medical scientist in the Ming Dynasty, proposed that silkworms have therapeutic effects on diabetes. Preliminary studies indicated that crude silkworm powder has a blood glucose-lowering effect in diabetic mice [2-5]. Another clinical study that had 38 type 2 diabetes mellitus (T2DM) patients treated with metformin and silkworm extract (SE) showed that the silkworm powder lowered postprandial blood glucose levels [6]. Unfortunately, it was not convenient for patients to administer the coarse powder orally, so to improve patient compliance, four types of SE were prepared with different polar solvents (ethanol, n-butanol, ethyl acetate and petroleum ether). The active fraction of SE in each solution was then screened in vitro, as shown in our previous studies [7]. The ethanol extract was the most effective in reversing insulin resistance in 3T3-L1 adipocytes while also ameliorating the damage induced by hydrogen peroxide in MIN6 cells derived from mouse pancreatic β-cells [7]. The in vitro analysis suggested that SE has a hypoglycemic effect; therefore, administering it to T2DM rats could further elucidate its mechanism of action and advance its clinical usage. In this study, we established a T2DM rat model induced with a high fat diet and streptozotocin (STZ). The blood glucose, free fatty acid (FFA), malondialdehyde (MDA), tumor necrosis factor alpha (TNF-α) and superoxide dismutase (SOD) levels were detected and histopathological examination and immunohistochemistry (IHC) staining were performed. The analyses revealed the beneficial effects of SE in T2DM.
The silkworm strain, Qingsong Haoyue, was provided by Fujian Provincial Silkworm Institute, Fujian Province, China. On the third day of the fifth-instar, silkworm larvae were collected and then frozen immediately at − 20 °C for 48 h. Subsequently, the frozen silkworms were dried at − 40 °C to ensure maximum moisture content of 4%. Before extraction with 70% (v/v) aqueous ethanol, the freeze-dried silkworms (5 kg) were defatted with 15 L petroleum ether (boiling range 60 - 90 °C) for 6 h at (24 ± 2) °C. After filtering under reduced pressure at 40 °C, SE was freeze-dried at − 55 °C using a freeze dryer (Savant Modulyo D Freeze dryer, Thermo Electron Corporation, America). Finally, 796 g SE powder (1 g of SE equals to 6.28 g of crude frozen-dried silkworm) was obtained and stored at 4 °C before being used; the percent yield was 15.9% (w/w).
SE samples were dissolved in a solvent (methanol : 25% HCl = 4 : 1) and filtered with a 0.45 μm syringe filter (Merck Millipore, Germany). The HPLC system (Agilent 1260, Agilent, USA) consisted of a G1311 quaternary pump, G1329B autosampler, degasser, G1316A1200 automatic thermostatic column compartment, and a G1315D diode array detector as well as a computer with Chemstation software (OpenLAB Data Analysis, M8370AA, Germany). The analytical column used was Eclipse XDB-C18 (5 μm, 4.6 mm × 250 mm, Agilent, USA) at 30 °C. Acetonitrile and 0.4% phosphoric acid were used as mobile phase in a gradient mode; it was degassed automatically using the electronic degasser system with 1.0 mL/min of flow rate. Diode-array detector was set at 360 nm and sample was injected in splitless mode. According to our previous study on silkworm active components [8], it contains multiple antioxidant flavonoids. In our present study, two antioxidant compounds (quercetin and kaempferide) were measured to control the silkworm quality, as described in a previously published study [9].
Six-week-old male SPF grade Sprague-Dawley (SD) rats purchased from Slrc Laboratory Animal Company (Laboratory animal quality certificate No. SCXK (HU) 2012-0002, Shanghai, China; Laboratory animal use permit No. SYXK (MIN) 2013-0006) were housed in an air-conditioned room with controlled temperature (24 ± 2) °C, humidity (55% ± 15%), and a 12 h light-dark cycle. After one week of acclimatization, rats in the normal control group were fed ordinary animal chow and the remaining groups were fed a high fat diet with 60 cal% fats (D12492, Research Diet, Inc., USA) to induce insulin resistance. Seven weeks later, the rats fed with a high fat diet were injected with a single low dose of STZ (40 mg/kg i.p. in 0.01 mol/L citrate buffer pH 4.5) to induce diabetes. One week later, non-fasting blood glucose (NFBG) levels were measured in all rats using a portable blood glucometer (Lifescan Inc., USA) through a tail vein puncture. Rats with NFBG > 11.1 mmol/L were considered diabetic [10].
All animals were randomly divided into five groups (n = 8) as follows: normal control (NC), diabetic model control (MC), model + low-dose of SE (SEL; 0.5 g/kg BW), model + high-dose of SE (SEH; 1 g/kg BW), and model + 20 mg/kg BW of pioglitazone (PC; Takeda Pharmaceuticals, USA). All rats of treatment groups were administered the drugs, while the rats in the normal control and diabetic model groups were treated with equal amounts distilled water. Body weight and NFBG in all groups were monitored every week. All animal experimental protocols used in this study were subject to approval by the Experimental Animal Research Ethics Committee of Xiamen Medicine Research Institute, Xiamen, China.
OGTT was conducted according to previously described methods [11]. During the last week, all rats were fasted for 12 h and gavaged glucose solution (2 g/kg BW). Blood glucose was measured at 0, 30, 60, 90 and 120 min after glucose ingestion, respectively.
At the end of the study, all rats were anesthetized with ethyl ether. Blood samples were collected via cardiac puncture, centrifuged at 1 000 × g for 15 min to obtain serum and stored at − 20 °C (Eppendorf Centrifuge 5810R, Germany). The pancreatic tissue of each animal was removed immediately and fixed with 10% neutral formalin buffer at room temperature.
TNF-α, FFA, SOD and MDA levels were measured using an enzyme-linked immunosorbent assay (ELISA). All operations were performed strictly in accordance to the kit instructions. Reagent kits (The LOT number of all ELISA kits is 10/2018.) were purchased from Nanjing Jiancheng Bioengineering Institute (Nanjing, China).
Hematoxylin-eosin (HE) staining was performed for histological evaluation. The pancreatic tissue was sliced (4 μm), deparaffinized in p-xylene, and rehydrated in gradient ethanol (100%, 80%, 70% and 50%). After gently rinsing with distilled water, the tissue slides were counter-stained with eosin, mounted in neutral balsam, and observed under a Leica microscope (Germany Leica Microsystems Co., Ltd., Germany).
Deparaffinized and rehydrated slides were immersed in distilled water. Antigen retrieval was carried out by heating slides at 80 °C in 10 mmol/L citrate buffer solution for 5 min. After washing three times with distilled water, 3.0% peroxide was used to block the activity of endogenous peroxidase. To avoid non-specific staining, slides were incubated with normal goat serum at room temperature for 20 min. The sections were then incubated in 5% Bovine albumin (BSA) for 1 h at room temperature, followed by treatment with rabbit polyclonal anti-insulin antibody (1 : 200; ab216418, Abcam, Cambridge, UK) overnight at 4 °C. Negative control sections were stained with equivalent concentrations of normal rabbit IgG under identical conditions. The slides were subsequently washed with phosphate buffered saline and subjected to a color reaction with freshly prepared diaminobenzidine. Brown particles or linear deposits were counted as positive areas.
Data are expressed as mean ± standard deviation (SD) and were analyzed using one-way analysis of variance and Tukey’s HSD test. Differences at P < 0.05 were considered statistically significant.
Figure 1 shows the chromatograms of the mixed standards (quercetin and kaempferide) and SE sample. In the HPLC fingerprints of SE sample, the peaks of quercetin and kaempferide were detected by matching their retention times with those of the standards. HPLC analysis revealed that the content of quercetin in silkworm powder was 3.217 mg/g and the content of kaempferol was 0.983 mg/g.
To test the effects of SE on body weight, rats in all groups were weighed before and after treatment. Compared with normal rats, body weight in the other groups did not change significantly during the first four weeks. However, it markedly increased in the next three weeks and decreased after STZ injection (P < 0.05, Figure 2). Body weight in all treatment groups (SE and pioglitazone) increased to near normal levels (P < 0.05) compared with that in the model group.
Figure 3 shows the hypoglycemic effect of SE on T2DM rats for various diet groups over a five-week period. NFBG in diabetic rats was significantly higher than that in normal rats (P < 0.05). Pioglitazone- and SE-treated rats had lower NFBG compared with T2DM rats (P < 0.05). 12 weeks after treatment, blood glucose levels of the SE group were similar to the PC group.
Figure 4 shows significantly higher blood glucose levels in the model group than any other groups (P < 0.05). Blood glucose peaked 30 min after administration followed by a decrease. The glucose-lowering effect of the PC and SEH group was the most significant (P < 0.05).
Compared with the normal group, TNF-α, FFA and MDA levels in the model group were significantly higher (P < 0.01). After pioglitazone and SE treatment, TNF-α, FFA and MDA levels decreased sharply (P < 0.01), while SOD levels increased (P < 0.01), as displayed in Figure 5.
The pancreatic histopathological analysis presented in Figure 6 revealed fat infiltration, reduced number of pancreatic β-cells, and islet atrophy in the model group. Interestingly, pancreatic β-cells increased significantly, while β-cell vacuole degeneration and inflammatory cell infiltration reduced after treatment, particularly in the SEH group.
Diabetes, considered to be the third largest non-infectious chronic disease in the world after cardiovascular diseases and cancer, severely impacts human health and occurs due to islet β-cell dysfunction. Records about diabetes treatment with silkworm in ancient medical books [12] have been long known. Silkworm’s pharmacological and hypoglycemic effects have also been demonstrated in modern research; however, its protective mechanisms on islet function and its active ingredients remain unclear [13]. Previous in vitro cell experiments from our laboratory [5] showed that the alcoholic SE not only promoted the proliferation of normal MIN6 cells, but also had a protective effect on H2O2-damaged MIN6 cells, further enhancing survival rate and inhibiting apoptosis. In the present study, a high fat diet combined with STZ were used to induce type 2 diabetes in rats to further investigate the hypoglycemic effects and active ingredients of SE, both in vivo and in vitro. Results showed that these anti-diabetic effects may be mediated by alleviating pancreatic inflammation and oxidative stress, preserving and repairing damaged islet cells, and reducing islet dysfunction.
Previous studies have demonstrated that inflammatory factor-mediated inflammation plays a critical role in diabetes development [14-16]. TNF-α is mainly secreted by macrophages and adipocytes in adipose tissues, sustaining mildly elevated levels under pathological conditions [14]. Under normal physiological conditions, phosphorylation of insulin receptor substrate (IRS) tyrosine further causes downstream multi-pathway signal transduction. TNF-α can induce insulin resistance (IR) and pancreatic islet β-cell apoptosis in various ways, ultimately leading to diabetes. It has been reported that TNF-α can suppress the interaction between the phosphatidylinositol 3-kinase (PI3-K) regulatory subunits and phosphorylated IRS by inhibiting PI3-K activity and disrupting downstream signal transduction [15]. NONG et al. [16] have shown that TNF-α indirectly fosters the phosphorylation of serine and threonine in IRS, hinders the normal tyrosine phosphorylation in IRS, and interferes with the insulin signal transduction process, eventually promoting IR. Furthermore, TNF-α can promote IRS degradation and islet β-cells apoptosis, resulting in oxidative stress. FFAs are potent cytotoxic active substances secreted by adipose tissue. Lipid toxicity, accompanied by damage to various tissues and cells as well as islet cells dysfunction and insulin resistance, is caused by persistently high levels of serum FFAs [17]. We found that the TNF-α and FFA levels in diabetic rats were significantly decreased by SE, suggesting that SE may improve insulin resistance by dampening the inflammatory reaction and facilitating the fatty acid re-esterification process.
Increased oxygen free radicals and imbalance between oxidation and anti-oxidation causes oxidative stress that can induce islet β-cell apoptosis and inhibit insulin secretion [18], reduce the sensitivity of peripheral tissues to insulin, and cause insulin resistance. MDA is a kind of lipid peroxide, and its content can be detected to reflect the degree of lipid peroxidation and oxidative damage [19]. MDA affects the key mitochondrial enzymes and respiratory chain complex thus promoting islet cell injury. This is attributed to changes in membrane permeability and mitochondrial swelling caused by impaired structure and function of the cell membrane [20]. SOD is an antioxidant that prevents accumulation of excessive levels of oxygen free radicals, and is a standard for measurement of antioxidant capacity [21]. Under pathological conditions, elevated MDA or lowered SOD levels may lead to insulin signal transduction inhibition or islet β-cells damage. This imbalance affects the signaling pathways related to insulin synthesis and secretion, thus inducing insulin resistance due to increased levels of reactive oxygen species (ROS) and pro-inflammatory cytokines that result from NF-κB pathway activation [22]. Taken together, inflammation and oxidative stress lead to insulin resistance and impaired pancreatic islet function, ultimately triggering diabetes. Our study showed that the SE treatment significantly increased SOD levels in diabetic rats, while decreasing MDA levels, indicating that it may treat diabetes by relieving oxidative stress.
The main active constituent in mulberry leaves and mulberry twig alkaloid tablets is the α-glucosidase inhibitor 1-Deoxynojirimycin (DNJ) [23, 24]. The silkworms that feed on mulberry leaves have enriched alkali, flavonoids and other active constituents [25]. In addition, we had previously determined that the antioxidant content in silkworms is higher than that in mulberry leaves, silkworm excrement, and rigid silkworm [8]. Therefore, the present study further proved that silkworm not only possesses α-glucosidase inhibitory activity, but also improves insulin resistance that may be related to the high content of antioxidant components. Study limitations include the lack of data on insulin secretion and related biochemical parameters and insufficient evidence for explaining SE hypoglycemic effects on a molecular level. Therefore, it is necessary to further investigate its mechanism of action in future research.
The present study suggests that SE has hypoglycemic as well as pancreatic protective effects. The observed pharmacological effects of ameliorating inflammation and oxidative stress are closely related to the decreased FFA, MDA and TNF-α level and the elevated SOD level in T2DM model rats.
This study was supported by the Xiamen City Health and Family Planning Commission and the Xiamen Municipal Bureau of Science and Technology. We thank for the funding support from the Leading Project of Fujian. P. R. C. (No. 2019D018) and the Xiamen Science and Technology Project (No. 3502Z20174058).
[1] |
Zhejiang Agricultural University. Anatomy and Physiology of Silkworm Body. Beijing: Agricultural Press, 1995: 4-9.
|
[2] |
RYU KS, LEE HS, CHUNG SH, et al. An activity of lowering blood-glucose levels according to preparative conditions of silkworm powder. Korean Journal Sericulture Science, 1997, 39(1): 79–85.
|
[3] |
RYU KS, LEE HS, KIM I. Effects and mechanisms of silkworm powder as a blood glucose-lowering agent. Journal of Sericultural and Entomological Science, 2002, 4(1): 93–100.
|
[4] |
ZHOU J, HAN D. Proximate, amino acid and mineral composition of pupae of the silkworm Antheraea pernyi in China. Journal of Food Composition & Analysis, 2006, 19(8): 850–853.
|
[5] |
ZHAO Y, WEI ZJ, JIANG ST, et al. Effects of silkworm eggs powder on SOD and SOD mRNA expression in diabetic mice. Food Science, 2007, 28(7): 466–469.
|
[6] |
KIM HJ, CHOI SW, CHO SH. Effects of various mulberry products on the blood glucose and lipid status of streptozotocin-induced diabetic rats. The Korean Journal of Nutrition, 2010, 43(6): 551. doi: 10.4163/kjn.2010.43.6.551
|
[7] |
ZHAO SF, XU GH, HUANG YQ, et al. Effect of Bombyx mori alcohol extract on the proliferation and apoptosis of MIN6 cells. Modern Distance Education of Chinese Medicine in China, 2018, 16(7): 97–99.
|
[8] |
QI HY, MA XY, XU GH, et al. Analysis of anti-oxidant components of silkworm. Strait Pharmaceutical Journal, 2020, 32(9): 35–39.
|
[9] |
ZHAO YL, HUANG YQ, HU SM, et al. Determination of quercetin and kaempferide in silkworm powder by HPLC. Chinese Journal of Experimental Traditional Medical Formulas, 2012, 18(18): 95–97.
|
[10] |
LU JM, WANG YF, YAN HL, et al. Antidiabetic effect of total saponins from Polygonatum kingianum in streptozotocin-induced diabetic rats. Journal of Ethnopharmacology, 2016, 179(2): 291–300.
|
[11] |
SALAHUDDIN M, JALALPURE SS. Antidiabetic activity of aqueous fruit extract of Cucumis trigonus Roxb in streptozotocin-induced-diabetic rats. Journal of Ethnopharmacology, 2010, 127(2): 560–567.
|
[12] |
(MING) LI SZ. Compendium of Materia Medica. Taiyuan: Shanxi Science and Technology Press, 2014.
|
[13] |
HE WQ, JIN J, SHI LG, et al. Advances in pharmacological research on active substances of silkworm larvae. Silkworm Mulberry, 2005, 36(2): 5–9.
|
[14] |
QI ZY, WEI AS, ZHANG SC. Effects of Modified Shashen Maidong Decoction on insulin resistance, inflammation and oxidative stress in diabetic rats. Journal of Guangzhou University of Traditional Chinese Medicine, 2019, 36(5): 724–728.
|
[15] |
GRIGSBY RJ, DOBROWSKY RT. Inhibition of ceramide production reverses TNF-induced insulin resistance. Biochemical and Biophysical Research Communication, 2001, 287(5): 1121. doi: 10.1006/bbrc.2001.5694
|
[16] |
NONG TL, ZENG HY. The relationship between TNF-α, IL-6, IL-8 and DM2. Journal of Radioimmunology, 2011, 24(2): 140–141.
|
[17] |
LI Z, SHEN M, LIU QH. Clinical application value of serum free fatty acids in the development of complications of type 2 diabetes. Journal of Taishan Medical College, 2020, 41(2): 106–108.
|
[18] |
WANG YQ, WU XL, YU XT. The protective effect of Liuhuang mixture on pancreatic islet β cells in insulin resistant rats. Advances in Anatomy, 2014, 20(2): 109–111.
|
[19] |
YAN Y, PANG GM, MIAO MS, et al. Effects of Huanglian Jiangtang Tablets on glucose and lipid metabolism and adiponectin in type 2 diabetic rats. Chinese Journal of Traditional Chinese Medicine, 2019, 34(11): 5415–5418.
|
[20] |
SZKUDELSKA K, NOGOWSKI L, SZKUDELSKI T. Resveratrol and genistein as adenosine triphosphate-depleting agents in fat cells. Metabolism, 2011, 60(5): 720–729. doi: 10.1016/j.metabol.2010.07.006
|
[21] |
KASZNICKI J, SLIWINSKA A, KOSMALSKI M, et al. Genetic polymorphisms (Pro197Leu of Gpx1, +35A/C of SOD1, -262C/Tof CAT), the level of antioxidant proteins (GPx1, SOD1, CAT) and the risk of distal symmetric polyneuropathy in Polishpatients with type 2 diabetes mellitus. Advances in Medical Science, 2016, 61(1): 123. doi: 10.1016/j.advms.2015.10.006
|
[22] |
COUDRIET, G M, DELMASTRO-GREENWOOD MM, PREVITE DM, et al. Treatment with a Catalytic Superoxide Dismutase (SOD) mimetic improves liver steatosis, insulin sensitivity, and inflammation in obesity-induced type 2 diabetes. Antioxidants (Basel, Switzerland), 2016, 6(4): 85.
|
[23] |
LIU SN, LIU Q, LIU YL, et al. Review of the research and development history of Sangzhi total alkaloid tablets (2): modern pharmacological concepts interpret the pharmacological characteristics and pharmacological mechanism of traditional Chinese medicine. Chinese Journal of Diabetes, 2020, 28(8): 635–640.
|
[24] |
WANG Y, LI JY. Research on anti-diabetic active ingredients of mulberry leaves. Anhui Medicine, 2016, 20(3): 425–428.
|
[25] |
ZHAO YL, HUANG YQ. The anti-diabetic active ingredient of silkworm and its pharmacological research progress. Chinese Medicine Bulletin, 2009, 8(2): 63–66.
|
1. | Anusha, S., Negi, P.S. Processing of silkworm (Bombyx mori) pupae waste and mealworm (Tenebrio molitor) larvae: Chemical characterization of extracts rich in anti-oxidant, anti-diabetic, and anti-obesity activity. Biomass Conversion and Biorefinery, 2025. DOI:10.1007/s13399-025-06630-5 |
2. | Han, Y.-M., Lee, D.-Y., Song, M.-Y. et al. Toxicological Evaluation Verifies the Safety of Oral Administration of Steamed Mature Silkworm Powder in Rats. Foods, 2024, 13(14): 2209. DOI:10.3390/foods13142209 |
3. | Gao, S., Qian, Z., He, Z. et al. Analysis of nutrients in Apriona germari and treatment of diarrhea in mice fed with insect powder. Biochemistry and Biophysics Reports, 2023, 33: 101368. DOI:10.1016/j.bbrep.2022.101368 |
4. | Li, X.-Q., Jia, S.-S., Yuan, K. et al. Hypoglycemic Effect of the N-Butanol Fraction of Torreya grandis Leaves on Type 2 Diabetes Mellitus in Rats through the Amelioration of Oxidative Stress and Enhancement of β -Cell Function. Genetics Research, 2022, 2022: 5648896. DOI:10.1155/2022/5648896 |