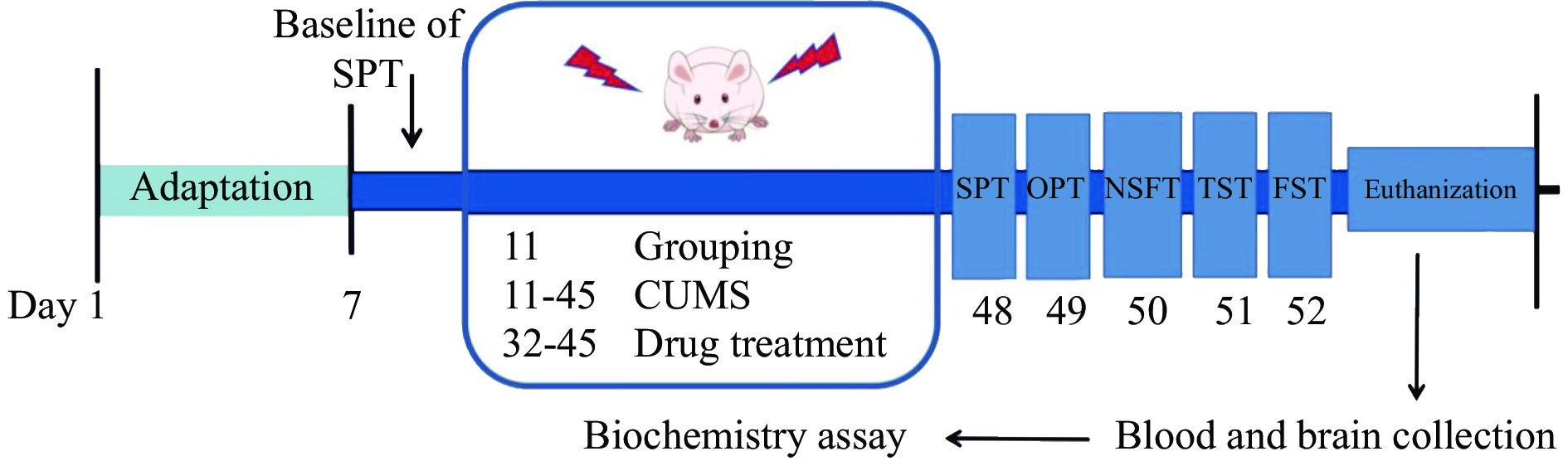
Citation: | Citation: CHEN YZ, ZHAO YZ, ZHANG YW, et al. Antidepressant effects of Yuanzhi (Polygalae Radix) extract on chronic unpredictable mild stress-induced depression in rats: modulation of the NLRP3 inflammasome and NF-κB pathway. Digital Chinese Medicine, 2024, 7(2): 184-194. DOI: 10.1016/j.dcmed.2024.09.009 |
To investigate the antidepressant effects of Yuanzhi (Polygalae Radix, PR) aqueous extract on chronic unpredictable mild stress (CUMS)-induced depression rat models and the underlying mechanisms.
A total of 40 male Sprague Dawley (SD) rats were randomly divided into control, model, low dose of PR (PR-L, 0.5 g/kg), high dose of PR (PR-H, 1 g/kg), and fluoxetine (10 mg/kg) groups, with 8 rats in each group. Except for the rats in control group, those in the other four groups underwent CUMS-induced depression modeling. PR and fluoxetine were administered intragastrically once daily, 30 min prior to the CUMS procedure, for 14 consecutive days until the behavioral tests were performed. After CUMS modeling, the sucrose preference test (SPT), open field test (OFT), novelty-suppressed feeding test (NSFT), forced swim test (FST), and tail suspension test (TST) were employed to assess the pharmacological effects of PR on the mitigation of depressive-like behaviors in rat models. Additionally, the enzyme-linked immunosorbent assay (ELISA) was utilized to quantify the serum levels of tumor necrosis factor (TNF)-α, interleukin (IL)-6, and IL-1β in the rats. Western blot analysis was also conducted to evaluate the protein expression levels of nuclear factor kappa-B (NF-κB), inducible nitric oxide synthase (iNOS), cyclooxygenase-2 (COX-2), nucleotide-binding oligomerization domain (NOD)-like receptor family pyrin domain containing 3 (NLRP3), apoptosis-associated speck-like protein containing caspase recruitment domain (ASC), and caspase-1 in the hippocampal tissues of the rats. Immunofluorescence staining was performed to observe the morphological changes in ionized calcium-binding adapter molecule 1 positive (Iba-1+) cells in the dentate gyrus (DG) of rats with CUMS-induced depression.
(i) Treatment with PR-H and fluoxetine resulted in significant enhancements in both the total distance and time the rats moved during tests (P < 0.01 and P < 0.05, respectively). Post-administration of PR-H and fluoxetine also led to statistically significant increase in sucrose preference among rats (P < 0.05). Besides, PR-L, PR-H, and fluoxetine treatment markedly decreased the latency of ingestion (P < 0.05, P < 0.05, and P < 0.01, respectively). As observed from the FST, PR-L, PR-H, and fluoxetine presented antidepressant effects on rats with CUMS-induced depression, leading to the reduction in time of their immobility (P < 0.05, P < 0.01, and P < 0.01, respectively). The results of TST indicated reduced immobility time in rats receiving PR-H and fluoxetine treatment as well (P < 0.01). (ii) Rats in model group showed an increase in the levels of Iba-1+ microglia in their left and right brains in comparison with control group (P < 0.01). However, such increase was negated post PR treatment (P < 0.01). Treatment with PR-L, PR-H, and fluoxetine considerably reduced the levels of inflammatory factors (TNF-α, IL-1β, and IL-6, P < 0.01). In addition, treatment of PR-L and PR-H effectively counteracted the elevated levels of NLRP3, ASC, and caspase-1, and markedly down-regulated the expression levels of phosphorylated p65 (p-p65), COX-2, and iNOS in rats’ hippocampus (P < 0.01).
Collectively, these findings indicate that PR exerts an antidepressant effect on rats with CUMS-induced depression partially through the modulation of the NLRP3 and NF-κB signaling pathways.
Depression is a multifaceted psychiatric condition that affects approximately 322 million people worldwide, making it one of the leading causes of mental health disability. It manifests as a variety of cognitive, emotional, and physical disorders characterized by negative emotions, diminished self-esteem, and cognitive sluggishness [1]. Furthermore, it is a significant risk factor for suicide, with approximately 90% of suicide cases showing a prominent link to the condition. Recent studies have indicated that the global prevalence of depression is around 4.4%, affecting an estimated 322 million individuals worldwide [2, 3]. Despite these statistics, the pathogenesis of depression remains unclear, significantly hindering the advancement of both antidepressant medications and clinical therapeutic strategies.
The complex pathophysiology of depression, particularly in response to psychological stress, has been extensively investigated, revealing that the immune system plays a significant role. There is robust evidence suggesting that microglial activation is associated with the occurrence of inflammation and has a pivotal impact on the onset and subsequent treatment of depression. The intricate relationship between these inflammatory processes and depression underscores the therapeutic potential of anti-inflammatory interventions [4]. Inflammation has long been recognized as a risk factor for depression-like behaviors in animals. Recent researches have uncovered specific mechanisms by which inflammation is implicated in the development of these behaviors [5, 6]. The prevailing hypothesis regarding proinflammatory cytokines suggests that inflammation significantly contributes to the onset of depression, with a reciprocal relationship where each condition exacerbates the other [7]. The activation of the nucleotide-binding oligomerization domain (NOD)-like receptor family pyrin domain containing 3 (NLRP3) inflammasome pathway, which triggers microglial activation, has been identified in the microglia cytoplasm. Upon perceiving stress, it assembles with apoptosis-associated speck-like protein containing caspase recruitment domain (ASC) and the precursors of caspase-1 to form the NLRP3 inflammasome that activates caspase-1 and releases inflammatory factors [8]. Moreover, the NLRP3 inflammasome plays a pivotal role in nuclear factor kappa-B (NF-κB)-mediated inflammatory responses, where it coregulates the transcription and activity of interleukin (IL)-1β, a key proinflammatory cytokine. Some studies have shown that among inflammatory markers, IL-6 exhibits the most robust correlation with depression, and it is significantly linked to the symptoms of anhedonia and psychomotor retardation [9]. The activation of NF-κB, which serves as a pivotal upstream regulator of proinflammatory cytokines, can induce apoptosis and lead to neuronal damage, highlighting its critical role in the neuroinflammatory process. Consequently, the inhibition of NF-κB activation not only suppresses the transcription of proinflammatory factors but also attenuates proinflammatory factor-induced disease symptoms [10].
Yuanzhi (Polygalae Radix, PR), the desiccated rootstock of Polygala tenuifolia Willd. or Polygala sibirica L., is predominantly utilized in traditional Chinese medicine as an expectorant, tonic, tranquillizer, and anti-psychotic drug. Insomnia, amnesia, and neurasthenia are some of the most common mental diseases for which PR is used as part of the treatment [11]. Furthermore, a growing body of research has highlighted the antidepressant and anti-inflammatory properties of PR, along with its key constituent compounds. Polygala saponins inhibit the NLRP3 inflammasome via SHP-2-mediated mitophagy in vitro and in vivo [12]. PR extract could modulate colonic inflammation, colonic microbiota, tight junctions, TPR-KYN metabolism, and NF-κB-NLRP3 signaling pathways to achieve a colonic balance within rats with chronic restraint stress-induced depression [13]. Recent study has reported that PR extract exhibits antidepressant effects in mice subjected to behavioral despair tests and rats exposed to chronic restraint stress, likely through the mechanisms of enhancing autophagy and inhibiting neuroinflammation [14]. However, the effects of PR on rat models with chronic unpredictable mild stress (CUMS)-induced depression have not been previously reported. Therefore, this study aims to investigate the effects of PR on CUMS-induced depression in rat models and to elucidate the underlying mechanisms. Utilizing various behavioral assays, including sucrose preference test (SPT), open field test (OFT), novelty-suppressed feeding test (NSFT), tail suspension test (TST), and forced swim test (FST), we systematically evaluated the antidepressant effects of PR on rats subjected to CUMS. Additionally, to elucidate the potential mechanisms underlying PR’s antidepressant action, we conducted assessments of neuroinflammatory markers, including neurotoxic mediators, microglial activation levels, and the expression of proinflammatory cytokines.
Fluoxetine hydrochloride (> 98%) was purchased from Patheon France Co., Ltd. (France). Pentobarbital sodium (P3761) was purchased from Sigma (USA). Commercial enzyme-linked immunosorbent assay (ELISA) kits for TNF-α, IL-6, and IL-1β were purchased from Nanjing Jiancheng Institute of Biotechnology (China).
A total of 40 specific pathogen-free (SPF) male Sprague Dawley (SD) rats, weighing 180 – 200 g, were supplied by Beijing Vital River Laboratory Animal Technology Co., Ltd. [SCXK (Jing) 2021-0006], with the batch number SLXD-20190314027. Five rats per cage were housed under standard experimental conditions, which included a controlled temperature of 25 ± 2 °C, a 12-hour light/dark cycle, and ad libitum access to food and water. The animals were allowed a one-week acclimation period to adjust to their new surroundings prior to any experimental procedures. All experimental procedures were conducted in strict compliance with the National Institutes of Health Guidelines for the Care and Use of Laboratory Animals. The study was approved by the Academy of Experimental Animal Center at the Institute of Medicinal Plant Development (SYXK 2017-0020).
The dehydrated roots of Polygala tenuifolia Willd. from the Polygalaceae family were utilized in our research. A herbal sample of PR from Yuncheng, Shanxi, China, was verified by Professor PAN Ruile of the Institute of Medicinal Plant Development (IMPLAD). A voucher specimen (registration number 20180512) was deposited in IMPLAD’s herbarium. PR of 1 000 g was subjected to three successive extractions with 8 L of water, each performed under reflux conditions for 1 h. Subsequently, the 1 000 mL extraction solution was concentrated using a rotary evaporator. The concentrated solution was then mixed with pure ethanol and refrigerated at 4 °C overnight to achieve a final concentration of 70% (w/w). The ethanol was subsequently removed from the filtrate under reduced pressure using the same rotary evaporator. To prepare the extract, PR was redissolved in 1 000 mL of water to reach a target concentration of 1 g/mL. For proper usage, the extract was diluted to the desired concentration at 20 °C.
Following acclimatization, the 40 rats were randomly divided into five groups (n = 8 in each group): control, CUMS-induced (model), CUMS-induced + 0.5 g/kg PR (PR-L), CUMS-induced + 1 g/kg PR (PR-H), and CUMS-induced + 10 mg/kg fluoxetine (fluoxetine) groups. The dosages of PR were determined based on previous study conducted in our laboratory [10]. Except for control group, all treatments were prepared by dissolving the drugs in distilled water and were administered intragastrically once daily, 30 min before the CUMS procedure, for a period of 14 d until the behavioral tests were performed. The experimental arrangement is as follows (Figure 1).
All groups, except for control group, were housed in individual cages and subjected to a 5-week CUMS treatment after acclimatization. This treatment was refined based on our previous study [15] and involved a variety of stressors (Table 1). Each day, two to three stressors were randomly applied to the rats, ensuring that the same stressor was not repeated for at least one week to prevent the rats from anticipating the onset of the stimulus. Control group was maintained in a separate room to avoid any interaction with those exposed to CUMS.
No. | Stressor | Time |
1 | Water deprivation | 24 h |
2 | Cage switching | 4 h |
3 | Illumination all night | 12 h |
4 | Predator sounds | 30 min |
5 | Soiled cage (200 mL water in 100 g sawdust bedding) |
12 h |
6 | Light/dark inverted | 24 h |
7 | Swimming in cold water (4 °C) | 5 min |
8 | Pinch tail | 3 min |
9 | Swimming in hot water (40 °C) | 5 min |
10 | Electrical stimulation | 3 min |
11 | The physical restraint | 6 h |
12 | Food deprivation | 24 h |
The body weight of each rat was recorded weekly, with measurements taken every Monday morning at 9:00 am. The impact of the CUMS-induced reaction on the rats’ body condition was assessed by comparing the changes in the body mass among the five groups at different time points.
Anhedonia, a primary feature of depression, can be reliably assessed by SPT, which serves as a valid indicator of hedonic behavior [16]. The SPT procedure was divided into the adaptation and test phases [17]. First, rats were housed in individual cages for 2 d to acclimate. During the initial 24 h, all rats received two water bottles: one containing a 1% sucrose solution and the other purified water. Throughout the adaptation phase, we alternated the position of the two bottles every 12 h. This approach aimed to prevent any potential preference bias and maintain a tranquil environment. The test entailed a 24 h deprivation of both food and water. Second, following a period of water and food deprivation, each rat received two pre-weighed bottles, one containing purified water and the other containing sucrose solution. The weights of the bottles were recorded before the test. And the bottles were weighed again 1 h after the test to determine the volumes of both purified water and sucrose solution consumed. The SPT was conducted both before and after the CUMS procedure. The percentage of sucrose preference was calculated using the formula: sucrose preference (%) = sucrose solution consumption/(sucrose solution consumption + purified water consumption) × 100%.
The test was routinely conducted to assess the spontaneous locomotor activity of rats and to evaluate the effects of antidepressant treatments [18]. Following a 1 h administration period, the rats were introduced into the open field apparatus, which measured 40 cm in height and 75 cm in diameter, and were allowed 5 min to explore freely. After each rat being tested, its feces were removed, and the apparatus were wiped with 10% alcohol. A computer-aided control system was utilized to monitor the rats’ locomotor activity, recording the total distance traveled and the duration of movement to evaluate their spontaneous activity levels.
The test was conducted according to a previous method [19]. It identifies behaviors indicative of depression and anxiety by measuring the latency of the rats’ feeding behaviors in response to novel factors [20]. The rats were food-deprived for 24 h prior to the test. Each rat was placed in a box, measuring 90 cm in diameter and 50 cm in height, with three pieces of standard rat chow positioned in the center. The rats were given 15 min to consume the chow ad libitum, and the onset of feeding, marked by the initial act of chewing, was meticulously recorded.
The test, as previously outlined by STERU et al. [21], involved suspending the rat approximately 70 cm above the floor using tape secured about 1 cm from the end of the tail. The rats were observed for a total duration of 6 min (criterion: rat’s tail in a straight line), and the duration of immobility was recorded during the last 4 min.
The animals were placed in a temperature-controlled swimming apparatus (height 30 cm, diameter 40 cm) with a water depth of 18 cm one day following the termination of TST, and the water temperature was maintained at 24 ± 1 °C [22]. On day 1 of the test, the rats were forced to swim for 15 min (training period). After a 24 h interval, the rats were reintroduced into the swimming apparatus for a 5 min test phase, during which they were allowed to swim freely. The duration of immobility, defined as the absence of any active swimming movements or struggling, was carefully recorded during this period.
Upon completion of the behavioral tests, the rats were euthanized with 10% ethyl carbamate. Blood samples were collected, and serum was isolated from the plasma by centrifugation at 3 500 r/min for 15 min at 4 °C. The isolated serum was then stored at – 80 °C for subsequent analysis. ELISA kits were employed to quantify proinflammatory cytokines, including TNF-α, IL-6, and IL-1β.
Hippocampal tissues were promptly excised, snap-frozen in radioimmunoprecipitation assay buffer (RIPA) supplemented with matched inhibitors, and homogenized. The resulting lysate underwent centrifugation at 12 000 r/min for 15 min at 4 °C to separate the phases. The supernatant was collected, and the protein concentration was determined using the bicinchoninic acid (BCA) protein assay kit (Solarbio, China). Subsequently, protein samples (30 – 50 μg) were electrophoresed on sodium dodecyl sulfate–polyacrylamide gel electrophoresis (SDS-PAGE) gels (8% – 15%) after denaturation and then transferred to polyvinylidene fluoride (PVDF) membranes (Millipore, USA). These membranes were blocked with 5% skim milk in Tris-buffered saline with Tween 20 (TBST) for 2 h, washed three times with TBST, and then incubated overnight at 4 °C with primary antibodies: phosphorylated p65 (p-p65) (1 : 2 000), p65 (1 : 2 000), NLRP3 (1 : 1 000), ASC (1 : 2 000), caspase-1 (1 : 1 000), and β-actin (1 : 2 000) (Cell Signaling Technology, USA), COX-2 (1 : 500), and iNOS (1 : 1 000) (USA), respectively. After thorough washing, the membranes were incubated with secondary antibodies comprising haptoglobin related protein (HPR)-goat anti-mouse IgG (1 : 5 000, Abcam, USA) at room temperature for 2 h. The enhanced chemiluminescence approach was used to detect protein expression, and imaging was performed with a ChemiDoc XRS instrument (Bio-Rad, USA). ImageJ software was employed for quantitative analysis. Data were normalized to the expression levels of β-actin, and the results are reported as percentages.
Following the completion of the behavioral tests, rats were thoroughly anaesthetized with sodium pentobarbital and underwent intracardial perfusion with 0.1 mol/L phosphate-buffered saline (PBS) supplemented with 4% paraformaldehyde. Brain tissue sections (6 μm) were collected and fixed with 4% paraformaldehyde fixative solution for 10 min. Goat serum albumin (5%) was used to prevent nonspecific binding sites. The specimen was incubated with rabbit anti-ionized calcium-binding adapter molecule 1 (Iba1) primary antibody (1 : 1 000) in PBS buffer for 60 min and then placed overnight at 4 °C. After washing three times with PBS, a diluted secondary antibody, tetramethylrhodamine isothiocyanate (TRITC)-conjugated goat anti-rabbit IgG (H + L) labelled with fluorophores was added dropwise, followed by incubation at room temperature for 2 h. The sections were counterstained with 4',6-diamidino-2-phenylindole (DAPI) (5 μg/mL) for 10 min. A Leica Q9 fluorescence microscope was employed to capture fluorescence images. ImageJ software was used for quantitative analysis.
Data analysis was conducted using SPSS 21.0. All data were expressed as mean ± standard error of the mean (SEM). The differences between the means were analyzed by one-way analysis of variance (ANOVA), followed by Tukey’s post hoc test. P < 0.05 was considered statistically significant.
Figure 2 illustrates that rats in control group exhibited greater body weight gain compared with all other groups during the 5-week investigation. From week 2 − 5, the body weight of control group was significantly higher than model group (P < 0.01). PR-L group exhibited a notable increase in weight compared with model group in week 4 and 5 (P < 0.05). In addition, PR-H showed a substantial weight increase compared with model group in week 5 (P < 0.01). Furthermore, fluoxetine group exhibited significant weight gain compared with model group during week 4 and 5 (P < 0.05).
To evaluate the effects of PR on depressive-like behaviors in rats, we conducted the SPT, OFT, FST, TST, and NSFT behavioral tests.
The results of OFT showed that model group exhibited significantly reduced values in total distance and movement time compared with control group (P < 0.01). However, treatment with PR-H and fluoxetine led to a substantial increase in both total distance and movement time (P < 0.01 and P < 0.05, respectively), indicating improved locomotor activity (Figure 3A – 3C).
The results of SPT showed that there were no substantial differences in sucrose preferences of rats in any group before the 5-week CUMS exposure. Post-CUMS exposure, model group showed a markedly reduced sucrose preference compared with control group (P < 0.01). However, subsequent treatment with PR-H and fluoxetine resulted in a significant enhancement in sucrose preference among the rats (P < 0.05, Figure 3D).
The results of NSFT results showed that the latency to initiate feeding was considerably prolonged in model group compared with control group (P < 0.05). However, after PR-L, PR-H, and fluoxetine treatment, the latency of ingestion markedly decreased (P < 0.05, P < 0.05, and P < 0.01, respectively, Figure 3E).
The results of FST showed that over the 5-week stimulation, model group exhibited a significant increase in immobility duration (P < 0.05). Administration of PR-L, PR-H, and fluoxetine elicited antidepressant effects, as evidenced by a reduction in immobility duration (P < 0.05, P < 0.01, and P < 0.01, respectively, Figure 3F).
Likewise, the CUMS increased the immobility time in the TST (P < 0.05), and PR-H and fluoxetine reversed this effect (P < 0.01, Figure 3G).
To explore the effects of PR on the neuroimmune response and microglial activation in rats with CUMS-induced depression, we investigated Iba-1+ cells in the dentate gyrus (DG). Iba-1+ microglia in control group displayed smaller cytostomes with scattered protrusions, while those in model group had larger cytostomes with thicker and more condensed protrusions. Following 14 d of PR-L, PR-H, and fluoxetine treatment, rats with CUMS-induced depression exhibited a remarkable reduction in activated microglial morphology (Figure 4A). Additionally the numbers of Iba-1+ microglia in both brain regions of model group rats significantly increased compared with control group (P < 0.01) . However, this increase was prevented by PR treatment (P < 0.01) (Figure 4B).
The ELISA results showed that the levels of proinflammatory cytokines TNF-α, IL-6, and IL-1β were markedly elevated in the hippocampal tissues of rats in model group compared with control group (P < 0.01, respectively). However, treatment with PR-L, PR-H, and fluoxetine considerably reduced the levels of these proinflammatory cytokines (P < 0.01, Figure 4C – 4E).
Figure 5 illustrates the protein expression levels of NLRP3, ASC, and caspase-1 in the hippocampus of rats, which were significantly higher in model group after 5 weeks of CUMS exposure compared with control group (P < 0.01). However, PR-L and PR-H administration effectively reversed this elevation (P < 0.01 and P < 0.05, respectively).
Subsequently, we explored whether the NF-κB pathway was connected to the antidepressant effects of PR in the hippocampus. NF-κB plays a pivotal role in the regulation of neuroinflammation, as this main transcription factor governs both microglial activation and inflammatory cytokine production. Western blot analysis revealed significantly elevated protein expression levels of p-p65, iNOS, and COX-2 in model group compared with control group (P < 0.01). However, PR treatment effectively reduced the expression levels of p-p65, COX-2, and iNOS (P < 0.01, Figure 6).
Depressive disorders are closely linked to both acute and chronic stressors. Increasing evidence has emphasized a positive correlation between depression and exposure to stressful events. Stress-exposed rat models are frequently employed in the study of depression, and the CUMS-exposed rat model is a typical one designed to simulate stressful events in human life, inducing depression-like symptoms in animals, including lack of exercises, reduced food and water consumption, and reduced responsiveness to rewarding stimuli [23, 24]. This depression model is widely recognized as highly valuable and promising due to its pathophysiological mechanisms, which closely resemble those of human depression [25]. Numerous studies have indicated that chronic stress causes emotional, cognitive, and memory impairments, as well as atrophy in the cortical and limbic regions of the brain and a reduction in hippocampal neuronal density [26-28]. Consistent with these findings, rats were subjected to 35 consecutive days of unpredictable mild stress, which resulted in the development of depressive behaviors. This procedure successfully induced key symptoms of depression and anhedonia, mirroring those observed in human patients with depressive disorders. Therefore, we chose CUMS-exposed rat models to investigate PR and the potential mechanisms of its antidepressant effect. In our research, five behavioral tests were employed to evaluate the depression models. Notably, the SPT, which measures typical clinical manifestations of depression, was employed to assess anhedonia [29]. It is a method designed based on the preferences of rats for sweetness. Following a lasting period, the animals were provided with plain water and sucrose water at low concentrations. The depressive symptom of anhedonia, characterized by a diminished ability to experience pleasure, was assessed by measuring the animals’ preference for sucrose [30]. The results of the TST and FST mainly reflect the degree of despair in rats, while the OFT assesses their exploratory behavior and curiosity [31, 32]. Our findings suggest that PR ameliorates depression-like behaviors, as evidenced by increased body weight, a higher sucrose preference index, enhanced performance in the OFT, shortened feeding latency in the NSFT, and decreased immobility in both the TST and FST.
Depression is intricately connected to inflammation, a condition often precipitated by a multitude of internal and external stressors. These stressors can lead to dysregulation of neurotransmitters and the hypothalamic-pituitary-adrenal axis, ultimately resulting in the development of anxiety and depressive disorders [33]. The activation of the NLRP3 pathway is crucial for the progression of neuroinflammation. Evidence has shown that the NLRP3 inflammasome, a complex assembly of proteins, significantly contributes to the pathogenesis of depression [34]. Primarily activated in response to various stressors, including psychological stress and neuroinflammatory stimuli, the NLRP3 inflammasome orchestrates a cascade of inflammatory responses that are implicated in depressive disorders. Upon activation, the NLRP3 inflammasome facilitates the cleavage of pro-caspase-1 into its active form, caspase-1 [35]. This activation initiates the maturation of pro-inflammatory cytokines, transforming IL-1β and IL-18 from their inactive precursors into their biologically active forms, which are crucial in mediating inflammatory responses. The release of these cytokines initiates a cascade of neuroinflammatory responses that contribute to the pathophysiology of depression. IL-1β and IL-18 are key mediators in the inflammatory response, leading to the disruption of synaptic plasticity, increased permeability of the blood-brain barrier, and enhanced microglial activation [36]. These events collectively result in neuroinflammation, which is closely linked to depressive symptoms. In this study, treatment with PR significantly reduced the expression levels of proinflammatory mediators including TNF-α, IL-1β, and IL-6, and down-regulated the protein expression levels of NLRP3, ASC, and caspase-1 in the rat hippocampus [37]. Furthermore, NF-κB is inactive in the cytoplasm under normal conditions, and activated NF-κB can upregulate NLRP3 expression level. NF-κB is a vital transcription factor regulating immune inflammation-related genes and consists of a p50-p65 heterodimer. Recent study has demonstrated a correlation between increased production of proinflammatory cytokines and depressive symptoms. Rats in a neuroinflammatory state exhibit behaviors that closely resemble those observed in depression [38]. After exposure to CUMS, the activation and phosphorylation of p65 lead to its translocation into the nucleus, where it enhances the production of proinflammatory cytokines. This increase results in the release of substantial proinflammatory factors, causing hippocampal neuronal damage [39]. In our study, we observed a significant increase in the expression level of NF-κB p65 in CUMS-exposed rats, which is consistent with previous studies. Importantly, PR treatment effectively reduced the activity of NF-κB.
Additionally, iNOS and COX-2 are two important signaling proteins in the NF-κB signaling pathway. The COX-2 promoter sequence contains a binding site that is specific to NF-κB. Upon binding to NF-κB and iNOS, this sequence facilitates the transcription of the COX-2 gene [40, 41]. The COX-2 gene is coordinated with damage to cellular DNA and proteins directly and acceleration of apoptosis of neuronal cells, contributing to depression. Study has shown that the activation of NF-κB and the expression level of iNOS are essential for the transcription of proinflammatory cytokines. This process could be linked to an increase in oxidative-nitrosative stress, which plays a significant role in the pathophysiology of inflammation and related disorders [42]. The inhibition of NF-κB and iNOS led to a decrease in cytokine expression and oxidative-nitrosative stress. Additionally, it mitigated inflammation-induced behavioral abnormalities [43]. Our findings align with previous research showing that CUMS leads to elevated levels of inflammatory cytokines and activation of the NLRP3 inflammasome. Treatment with PR effectively attenuated the activation of both the NLRP3 and NF-κB pathways. Notably, PR demonstrated strong antidepressant effects, as well as significant anti-inflammatory and neuroprotective properties. Therefore, PR emerges as a promising therapeutic agent for preventing the occurrence of depression.
The central nervous system relies on glial cells, specifically microglia and astrocytes, as the primary components of its innate immune defense [44, 45]. Microglia, a specialized type of macrophage, play a crucial role in the pathogenesis of depression. They are the primary orchestrators of the inflammatory response within the brain. In animal models of depression, deficits in synaptic plasticity and the activation of microglia are concurrent with increased levels of proinflammatory cytokines in both the peripheral blood and the brain [46, 47]. Recent research has highlighted that M1-like microglial activation, marked by increased expression of iNOS and proinflammatory mediators, plays a critical role in driving inflammation. Moreover, an increased density of glial cells, particularly in the cornu ammonis and dentate gyrus regions of the hippocampus, has been observed in patients with depression [48]. Iba-1 serves as a specific marker of microglial activation. Immunofluorescence analysis demonstrated that the fluorescence intensity of Iba-1 in the DG area was significantly higher in model group compared with control group. However, different doses of PR effectively suppressed microglial activation. Overall, our findings suggest that PR exerts its antidepressant effects through its anti-inflammatory properties.
In summary, the results indicate that PR can effectively alleviate depression-like behavior in rats with CUMS-induced depression. Additionally, PR can enhance anti-inflammatory responses in rats by modulating the NLRP3 and NF-κB pathways, as well as regulating inflammatory factors and microglial activation. This study adds to the growing body of evidence supporting the efficacy of PR in treating depression. While the findings of this study contribute valuable insights into the antidepressant and anti-inflammatory effects of PR in a rat model, several limitations should be acknowledged. The study primarily focuses on the NLRP3 and NF-κB pathways, yet the underlying mechanisms of PR's effects might involve other biological pathways and processes that were not explored in this research. A more comprehensive analysis of additional molecular targets and signaling pathways could provide deeper insights of the therapeutic potential of PR.
PR exerts antidepressant effects in CUMS-exposed rat models partially by inhibiting proinflammatory cytokines and microglial activation. These effects may be mediated through modulation of the NLRP3 and NF-κB pathways.
International Cooperative Project of Traditional Chinese Medicine (GZYYG2020023), CAMS Innovation Fund for Medical Sciences (CIFMS) Grant (2021-I2M-1-034), and Key Research Project of Hunan Province (222SK2018).
Competing Interests: The authors declare no conflict of interest
[1] |
MCCARRON RM, SHAPIRO B, RAWLES J, et al. Depression. Annals of Internal Medicine, 2021, 174(5): ITC65–ITC80. doi: 10.7326/AITC202105180
|
[2] |
SLAVICH GM, SACHER J. Stress, sex hormones, inflammation, and major depressive disorder: extending social signal transduction theory of depression to account for sex differences in mood disorders. Psychopharmacology, 2019, 236(10): 3063–3079. doi: 10.1007/s00213-019-05326-9
|
[3] |
MCGRATH JJ, AL-HAMZAWI A, ALONSO J, et al. Age of onset and cumulative risk of mental disorders: a cross-national analysis of population surveys from 29 countries. The Lancet Psychiatry, 2023, 10(9): 668–681. doi: 10.1016/S2215-0366(23)00193-1
|
[4] |
CHEN Z, KAMEI C. Facilitating effects of histamine on spatial memory deficit induced by scopolamine in rats. Acta Pharmacologica Sinica, 2000, 21(9): 814–818.
|
[5] |
DOWLATI Y, HERRMANN N, SWARDFAGER W, et al. A meta-analysis of cytokines in major depression. Biological Psychiatry, 2010, 67(5): 446–457. doi: 10.1016/j.biopsych.2009.09.033
|
[6] |
LIU JJ, WEI YB, STRAWBRIDGE R, et al. Peripheral cytokine levels and response to antidepressant treatment in depression: a systematic review and meta-analysis. Molecular Psychiatry, 2020, 25(2): 339–350. doi: 10.1038/s41380-019-0474-5
|
[7] |
BEUREL E, TOUPS M, NEMEROFF CB. The bidirectional relationship of depression and inflammation: double trouble. Neuron, 2020, 107(2): 234–256. doi: 10.1016/j.neuron.2020.06.002
|
[8] |
QIN ZS, SHI DD, LI WQ, et al. Berberine ameliorates depression-like behaviors in mice via inhibiting NLRP3 inflammasome-mediated neuroinflammation and preventing neuroplasticity disruption. Journal of Neuroinflammation, 2023, 20(1): 54. doi: 10.1186/s12974-023-02744-7
|
[9] |
GUO BQ, ZHANG MY, HAO WS, et al. Neuroinflammation mechanisms of neuromodulation therapies for anxiety and depression. Translational Psychiatry, 2023, 13(1): 5. doi: 10.1038/s41398-022-02297-y
|
[10] |
LAWRENCE T. The nuclear factor NF-κB pathway in inflammation. Cold Spring Harbor Perspectives in Biology, 2009, 1(6): a001651. doi: 10.1101/cshperspect.a001651
|
[11] |
LIU MH, WANG X, GAO DJ. Polygalae Radix: review of metabolites, pharmacological activities and toxicology. Frontiers in Pharmacology, 2024, 15: 1420853. doi: 10.3389/fphar.2024.1420853
|
[12] |
QIU WQ, AI W, ZHU FD, et al. Polygala saponins inhibit NLRP3 inflammasome-mediated neuroinflammation via SHP-2-mediated mitophagy. Free Radical Biology and Medicine, 2022, 179: 76–94. doi: 10.1016/j.freeradbiomed.2021.12.263
|
[13] |
LI CC, YE F, XU CX, et al. Effect of Radix Polygalae extract on the colonic dysfunction in rats induced by chronic restraint stress. Journal of Ethnopharmacology, 2022, 294: 115349. doi: 10.1016/j.jep.2022.115349
|
[14] |
ZHOU YF, YAN MZ, PAN RL, et al. Radix Polygalae extract exerts antidepressant effects in behavioral despair mice and chronic restraint stress-induced rats probably by promoting autophagy and inhibiting neuroinflammation. Journal of Ethnopharmacology, 2021, 265: 113317. doi: 10.1016/j.jep.2020.113317
|
[15] |
JIANG N, WANG HX, LI CC, et al. The antidepressant-like effects of the water extract of Panax ginseng and Polygala tenuifolia are mediated via the BDNF-TrkB signaling pathway and neurogenesis in the hippocampus. Journal of Ethnopharmacology, 2021, 267: 113625. doi: 10.1016/j.jep.2020.113625
|
[16] |
LIU MY, YIN CY, ZHU LJ, et al. Sucrose preference test for measurement of stress-induced anhedonia in mice. Nature Protocols, 2018, 13(7): 1686–1698. doi: 10.1038/s41596-018-0011-z
|
[17] |
WANG W, ZHENG LL, XU LJ, et al. Pinocembrin mitigates depressive-like behaviors induced by chronic unpredictable mild stress through ameliorating neuroinflammation and apoptosis. Molecular Medicine, 2020, 26(1): 53. doi: 10.1186/s10020-020-00179-x
|
[18] |
MAES M, BERK M, GOEHLER L, et al. Depression and sickness behavior are Janus-faced responses to shared inflammatory pathways. BMC Medicine, 2012, 10: 66. doi: 10.1186/1741-7015-10-66
|
[19] |
BLASCO-SERRA A, GONZÁLEZ-SOLER EM, CERVERA-FERRI A, et al. A standardization of the Novelty-Suppressed Feeding Test protocol in rats. Neuroscience Letters, 2017, 658: 73–78. doi: 10.1016/j.neulet.2017.08.019
|
[20] |
SHEPHARD RA, BROADHURST PL. Hyponeophagia and arousal in rats: effects of diazepam, 5-methoxy-N, N-dimethyltryptamine, d-amphetamine and food deprivation. Psychopharmacology, 1982, 78(4): 368–372. doi: 10.1007/BF00433744
|
[21] |
STERU L, CHERMAT R, THIERRY B, et al. The tail suspension test: a new method for screening antidepressants in mice. Psychopharmacology, 1985, 85(3): 367–370. doi: 10.1007/BF00428203
|
[22] |
PORSOLT RD, BERTIN A, JALFRE M. Behavioral despair in mice: a primary screening test for antidepressants. Archives Internationales de Pharmacodynamie et de Therapie, 1977, 229(2): 327–336.
|
[23] |
FORBES NF, STEWART CA, MATTHEWS K, et al. Chronic mild stress and sucrose consumption: validity as a model of depression. Physiology & Behavior, 1996, 60(6): 1481–1484. doi: 10.1016/s0031-9384(96)00305-8
|
[24] |
CHAI HH, LIU B, ZHAN HQ, et al. Antidepressant effects of rhodomyrtone in mice with chronic unpredictable mild stress-induced depression. The International Journal of Neuropsychopharmacology, 2019, 22(2): 157–164. doi: 10.1093/ijnp/pyy091
|
[25] |
ANTONIUK S, BIJATA M, PONIMASKIN E, et al. Chronic unpredictable mild stress for modeling depression in rodents: meta-analysis of model reliability. Neuroscience & Biobehavioral Reviews, 2019, 99: 101–116.
|
[26] |
PIZZAGALLI DA. Depression, stress, and anhedonia: toward a synthesis and integrated model. Annual Review of Clinical Psychology, 2014, 10: 393–423. doi: 10.1146/annurev-clinpsy-050212-185606
|
[27] |
MILLER AH, MALETIC V, RAISON CL. Inflammation and its discontents: the role of cytokines in the pathophysiology of major depression. Biological Psychiatry, 2009, 65(9): 732–741. doi: 10.1016/j.biopsych.2008.11.029
|
[28] |
DUMAN RS. Neurobiology of stress, depression, and rapid acting antidepressants: remodeling synaptic connections. Depression and Anxiety, 2014, 31(4): 291–296. doi: 10.1002/da.22227
|
[29] |
MARKOV DD. Sucrose preference test as a measure of anhedonic behavior in a chronic unpredictable mild stress model of depression: outstanding issues. Brain Sciences, 2022, 12(10): 1287. doi: 10.3390/brainsci12101287
|
[30] |
HASEGAWA H, TOMITA H. Assessment of taste disorders in rats by simultaneous study of the two-bottle preference test and abnormal ingestive behavior. Auris Nasus Larynx, 1986, 13: S33–S41. doi: 10.1016/S0385-8146(86)80032-3
|
[31] |
CRYAN JF, MOMBEREAU C, VASSOUT A. The tail suspension test as a model for assessing antidepressant activity: review of pharmacological and genetic studies in mice. Neuroscience and Biobehavioral Reviews, 2005, 29(4/5): 571–625. doi: 10.1016/j.neubiorev.2005.03.009
|
[32] |
KATZ RJ. Animal models and human depressive disorders. Neuroscience & Biobehavioral Reviews, 1981, 5(2): 231–246.
|
[33] |
JIANG N, WEI SS, ZHANG YW, et al. Protective effects and mechanism of Radix polygalae against neurological diseases as well as effective substance. Frontiers in Psychiatry, 2021, 12: 688703. doi: 10.3389/fpsyt.2021.688703
|
[34] |
KAUFMANN FN, COSTA AP, GHISLENI G, et al. NLRP3 inflammasome-driven pathways in depression: clinical and preclinical findings. Brain, Behavior, and Immunity, 2017, 64: 367–383. doi: 10.1016/j.bbi.2017.03.002
|
[35] |
ALCOCER-GÓMEZ E, DE MIGUEL M, CASAS-BARQUERO N, et al. NLRP3 inflammasome is activated in mononuclear blood cells from patients with major depressive disorder. Brain, Behavior, and Immunity, 2014, 36: 111–117. doi: 10.1016/j.bbi.2013.10.017
|
[36] |
ZHANG Y, TAO MH, CHEN CY, et al. BAFF blockade attenuates DSS-induced chronic colitis via inhibiting NLRP3 inflammasome and NF-κB activation. Frontiers in Immunology, 2022, 13: 783254. doi: 10.3389/fimmu.2022.783254
|
[37] |
LI SS, FANG YQ, ZHANG YH, et al. Microglial NLRP3 inflammasome activates neurotoxic astrocytes in depression-like mice. Cell Reports, 2022, 41(4): 111532. doi: 10.1016/j.celrep.2022.111532
|
[38] |
LIU P, HU Y, GUO DH, et al. Potential antidepressant properties of Radix Polygalae (Yuan Zhi). Phytomedicine, 2010, 17(10): 794–799. doi: 10.1016/j.phymed.2010.01.004
|
[39] |
WU Z, ZHU AQ, TAKAYAMA F, et al. Brazilian green propolis suppresses the hypoxia-induced neuroinflammatory responses by inhibiting NF-κB activation in microglia. Oxidative Medicine and Cellular Longevity, 2013, 2013: 906726. doi: 10.1155/2013/906726
|
[40] |
LI RP, ZHAO D, QU R, et al. The effects of apigenin on lipopolysaccharide-induced depressive-like behavior in mice. Neuroscience Letters, 2015, 594: 17–22. doi: 10.1096/fj.03-0329fje
|
[41] |
JUNG YJ, ISAACS JS, LEE SM, et al. IL-1β-mediated up-regulation of HIF-1α via an NF-κB/COX-2 pathway identifies HIF-1 as a critical link between inflammation and oncogenesis. The FASEB Journal, 2003, 17(14): 2115–2117.
|
[42] |
GAŁECKI P, GAŁECKA E, MAES M, et al. The expression of genes encoding for COX-2, MPO, iNOS, and sPLA2-IIA in patients with recurrent depressive disorder. Journal of Affective Disorders, 2012, 138(3): 360–366. doi: 10.1016/j.jad.2012.01.016
|
[43] |
MYINT AM, STEINBUSCH HWM, GOEGHEGAN L, et al. Effect of the COX-2 inhibitor celecoxib on behavioural and immune changes in an olfactory bulbectomised rat model of depression. Neuroimmunomodulation, 2007, 14(2): 65–71. doi: 10.1159/000107420
|
[44] |
KIM YJ, HWANG SY, OH ES, et al. IL-1β, an immediate early protein secreted by activated microglia, induces iNOS/NO in C6 astrocytoma cells through p38 MAPK and NF-κB pathways. Journal of Neuroscience Research, 2006, 84(5): 1037–1046. doi: 10.1002/jnr.21011
|
[45] |
SHAO BZ, XU ZQ, HAN BZ, et al. NLRP3 inflammasome and its inhibitors: a review. Frontiers in Pharmacology, 2015, 6: 262.
|
[46] |
TREMBLAY MÈ, STEVENS B, SIERRA A, et al. The role of microglia in the healthy brain. The Journal of Neuroscience, 2011, 31(45): 16064–16069. doi: 10.1523/JNEUROSCI.4158-11.2011
|
[47] |
YIRMIYA R, RIMMERMAN N, RESHEF R. Depression as a microglial disease. Trends in Neurosciences, 2015, 38(10): 637–658. doi: 10.1016/j.tins.2015.08.001
|
[48] |
KAUR C, SIVAKUMAR V, ZOU ZR, et al. Microglia-derived proinflammatory cytokines tumor necrosis factor-α and interleukin-1β induce Purkinje neuronal apoptosis via their receptors in hypoxic neonatal rat brain. Brain Structure & Function, 2014, 219(1): 151–170. doi: 10.1007/s00429-012-0491-5
|
No. | Stressor | Time |
1 | Water deprivation | 24 h |
2 | Cage switching | 4 h |
3 | Illumination all night | 12 h |
4 | Predator sounds | 30 min |
5 | Soiled cage (200 mL water in 100 g sawdust bedding) |
12 h |
6 | Light/dark inverted | 24 h |
7 | Swimming in cold water (4 °C) | 5 min |
8 | Pinch tail | 3 min |
9 | Swimming in hot water (40 °C) | 5 min |
10 | Electrical stimulation | 3 min |
11 | The physical restraint | 6 h |
12 | Food deprivation | 24 h |